|
MAIN PAGE
> Back to contents
Security Issues
Reference:
Degterev A.K.
Assesment of the risk of degazation of the Black Sea: type of limnological disaster on lake Nyos reconsidered
// Security Issues.
2023. ¹ 4.
P. 69-77.
DOI: 10.25136/2409-7543.2023.4.69339 EDN: GVCACD URL: https://en.nbpublish.com/library_read_article.php?id=69339
Assesment of the risk of degazation of the Black Sea: type of limnological disaster on lake Nyos reconsidered
Degterev Andrey Kharitonovich
Professor, Department of Radioecology and Environmental Compliance, Sevastopol State University
299033, Russia, Sevastopol', g. Sevastopol', ul. Kurchatova, 7
|
degseb@yandex.ru
|
|
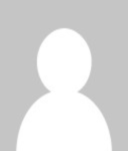 |
Other publications by this author |
|
|
DOI: 10.25136/2409-7543.2023.4.69339
EDN: GVCACD
Received:
12-12-2023
Published:
19-12-2023
Abstract:
The possibility of a repetition of the limnological catastrophe in the Black Sea is considered. It is shown that, in contrast to the volcanic lakes of Africa Nyos and Manun, where limnological catastrophes occurred in the 1980s, the concentration of dissolved gases in deep waters is significantly less than the saturation value. This eliminates the mechanism of so called “eruption” of carbon dioxide such as gas lift. However, on a smaller scale, significant methane releases are possible during strong eruptions of underwater mud volcanoes. The mechanism for the release of carbon dioxide from the lake into the atmosphere is so similar to a volcanic eruption that mathematical models developed for ordinary volcanoes are used to describe it. In both cases, the rise of erupted masses occurs due to an increase in the buoyancy of the gas-liquid mixture, which carries with it particles of the environment. The formation and growth of gas bubbles at intermediate depths occurs provided that the total partial pressure of all gases inside the bubble exceeds the hydrostatic pressure at a given depth. The article shows that the concentration of dissolved methane in the Black Sea is much less than the saturation level. Due to the relatively low solubility of methane in water, methane bubbles are able to overcome a significant depth range. And as additional components of the gas mixture, together with methane, carbon dioxide and hydrogen sulfide can thus enter the atmosphere. It was concluded that as the water temperature in the Black Sea increases due to climate change, the reserves of methane gas hydrate at the bottom of the sea will begin to decompose, which will also be accompanied by jet gas release. At the same time, methane can escape to the surface from depths of no more than 900 m.
Keywords:
limnological disaster, Lake Nyos, Black Sea, dissolved gases, carbon dioxide poisoning, gas bubbles, mud volcanoes, deep waters, disaster forecasting, natural disasters
This article is automatically translated.
You can find original text of the article here.
Introduction After two well-known disasters related to the release of dissolved carbon dioxide by volcanic lakes in Africa in the mid-1980s, the problem of the potential danger of meromictic reservoirs is very relevant. One of them occurred on the night of August 21, 1986, when approximately 1 km3 of carbon dioxide was released from Lake Nyos in the form of a 100 m high fountain of gas-water mixture, as a result of which 1,746 people and 3,500 cattle died within a few hours from CO2 poisoning within a radius of 30 km from the lake [4, 5, 14]. In this case, the source of the increased CO2 content in the water was the seepage of volcanic gases through the bottom for many years. The largest body of water with difficult vertical exchange is the Black Sea, and its depth is 2000 m, which is an order of magnitude greater than that of Lake Nyos. The solubility of carbon dioxide is proportional to the depth of reservoirs, which ensures the accumulation of a large amount of dissolved carbon dioxide in deep waters. It is also important that the solubility of carbon dioxide strongly depends on the water temperature (Table 1). Given that in the deep waters of the Black Sea it is 9oC, and in Lake Nyos 25oC, 1 liter of water in the case of the Black Sea may contain 72% more CO2 in the form of dissolved gas, while the same partial pressure. On the other hand, the solubility of gases in seawater is slightly less than in fresh water. Another condition for a possible "limnological eruption" of carbon dioxide by a reservoir is the presence of outlets of this gas at the bottom of the reservoir. For the Black Sea, and it is observed, numerous "sips" are known [1, 3, 7] in the form of upward-rising bubble "clouds", also called "torches". In addition, mud volcanoes at the bottom of the Black Sea have been well studied, the effect of which is associated with significant gas emissions. It is well known about the high content of carbon dioxide in groundwater on the coast. The mechanism of their entry into the atmosphere, as in the case of African lakes, may well be associated with the formation of bubble plumes at intermediate depths [13]. This is confirmed by the massive release of methane during the Crimean earthquake of 1927, when "gorenje seine" was observed with the formation of individual columns and entire bands of flashes near the coast of Crimea [7]. Thus, the analysis of the "eruption of carbon dioxide" by the Nyos and Manun lakes, taking into account their subsequent monitoring and the results of the measures taken to degass deep waters, is of undoubted interest in relation to the Black Sea. Dissolution of CO2 in water As is known, hydrogen sulfide and carbon dioxide have the highest solubility in water among all gases (Table. 1) [6]. And although hydrogen sulfide has twice the solubility, its content in volcanic gases is an order of magnitude lower. In any case, the concentration of both these dissolved gases in the deep waters of the Black Sea is about 10 mg/l, and it is slightly higher for CO2. Note that this value does not exceed 1% of the solubility of these gases, even at a pressure of 1 atm. This means, in particular, that the formation of bubbles from these gases due to degassing of the Black Sea waters is impossible even in surface waters. The fact is that hydrogen sulfide is still much more toxic (for the content of its MPC in drinking water = 0.03 mg / l), which has led to numerous speculations about the danger of hydrogen sulfide coming to the surface. In fact, the higher solubility of the gas in water leads to its greater dissolution when a bubble with a multicomponent gas mixture pops up, as a result of which, upon reaching the surface, its relative content in the mixture decreases very significantly [3]. Table 1 The solubility of gases in water (g/l) at a pressure of 1 atm. Temperature, oS | Oxygen | Carbon dioxide | Hydrogen sulfide | Methane | 10 20 30 | 0,054
0,043 0,036 | 2,32 1,69 1,25 | 5,11 3,93 3,00 | 0,030 0,024 0,020 | The danger of carbon dioxide release has also been underestimated so far because dissolved carbon dioxide itself accounts for only a few percent of the carbon dioxide dissolved in water. The fact is that the process of binding carbon dioxide to water includes the formation of a so-called carbonate system, which, in addition to the gas itself in solution, also includes the formation of bicarbonate ions, carbonate ions and carbonic acid molecules. It is these ions that account for most of the concentration of total dissolved inorganic carbon [6], which is about 2 mmol/l even in surface marine waters. And this corresponds to 90 mg/l in terms of carbon dioxide. At the same time, the concentration of dissolved carbon dioxide itself is about 0.1 mmol/l, that is, 4 mg/l. Dangerous concentrations of CO2 in the atmosphere Anatomical examination of the victims of the disaster at Lake Nyos in August 1986 revealed the presence of carbon dioxide in their lungs, which was the basis for the conclusion that they died from carbon dioxide poisoning. Although a number of witnesses who survived at that time mentioned the smell of hydrogen sulfide that night, which indicates its presence in the gas mixture in trace content. Analysis of dissolved gases in the deep waters of volcanic lakes in Cameroon and Rwanda showed that methane concentrations are also noticeable in them [12]. In Lake Nyos, its molar content reaches only 0.2 mmol/l, and in Lake Kivu it is over 20 mmol/l (Table 2). But methane is lighter than air, unlike carbon dioxide, which does not allow it to accumulate in large quantities in relief depressions. The proximity of the total pressure of dissolved gases to the saturation level at these depths indicates a high probability of lake degassing. Table 2 The content of dissolved gases in the deep waters of African lakes (mmol/kg) [12] Lake | Depth, m | Carbon dioxide gas | Methane | Nitrogen | Level saturation, % | Nyos |
171 | 132 | 0,272 | 0,166 | 24 | Manun | 69 | 128 | 1,65 | 0,140 | 67 | Kivu | 400 | 58 | 22 | 0,340 | 45 | The concentration of CO2 in the air of less than 0.5% is considered non-hazardous if you stay in such an atmosphere for no more than 8 hours a day. At its concentration of 5%, breathing becomes difficult, headache appears, pulse quickens, and a slight narcotic effect is manifested. You can only stay with her for a few minutes. If you are in an atmosphere with a CO2 concentration of 10%, then after 10-15 minutes loss of consciousness occurs. And already at concentrations above 15%, a rapid fatal outcome occurs, which is preceded by coma and convulsions. To estimate the probability of death from carbon dioxide poisoning, an empirical function P(C, t) is proposed, where C is the concentration of CO2 as a percentage and t is the time in minutes:
P = 0.5[1 + erf(C – ?)/?2 ?]. Here ? = 5.06 + 17.9/(1+ t0.36) and ? = 0.66 + 2.42/(1+ t0.35). It should be noted that the CO2 cloud in the vicinity of Lake Nyos was located for 2-4 hours, while hundreds of people who were in the valley at a distance of 20 km from the lake died. The peculiarity of CO2 poisoning is that prolonged exposure to an atmosphere with a concentration of carbon dioxide of only a few percent can lead to death. There is also information that even at such concentrations internal combustion engines do not work well, an open fire goes out [14]. Naturally, fish and many other marine organisms also die when a CO2 cloud forms above the surface of the water. The mechanism of the eruption In English, a limnological catastrophe is written as Lake eruption, that is, "lake eruption". And in fact, the mechanism of carbon dioxide release from the lake into the atmosphere was so similar to a volcanic eruption that mathematical models developed for ordinary volcanoes are used to describe it [11]. In both cases, the rise of the erupted masses occurs due to an increase in the buoyancy of the gas-liquid mixture, which carries with it particles of the environment. According to estimates [15], CO2 was released downwards from the lake in the form of a fountain of a gas-water mixture over 100 m above the surface of the lake. At the same time, large drops of water immediately fell back into the lake, and smaller ones were carried away by the resulting cloud of carbon dioxide in the direction of the wind and in the direction of lowering the relief. This is confirmed by the fact that the water level in the lake immediately after the disaster was 1 m lower. This is completely analogous to how an ordinary volcanic eruption occurs, only instead of water droplets, magma droplets are transferred in this way, which, when solidified, turn into a substance that is commonly called volcanic ash. There is also degassing of liquid magma. At the same time, the intake of carbon dioxide is so significant that it averages hundreds of million tons per year on the planet. In this sense, meromictic reservoirs play the role of a hearth under a volcano, where CO2 reserves accumulate, which subsequently triggers an eruption of the lake in the form of a fountain of gas-liquid mixture above its surface. And the movement of the CO2 cloud along the depressions of the terrain can be compared to a lava flow. As for the connection of the limnological catastrophe with the melting of carbon dioxide gas hydrates at the bottom of the reservoir, they are not there. CO2 hydrate can only be formed at water temperatures below 10 °C, which excludes the presence of its reserves at the bottom of lakes in Cameroon and Rwanda. Formation of a plume of bubbles in water The formation and increase of gas bubbles at intermediate depths occurs under the condition that the total partial pressure of all gases inside the bubble exceeds the hydrostatic pressure at a given depth z: ? Pi + 2?/r > Ratm + pgz , ( 1 ) where p is the density of water and ? is the coefficient of surface tension of water. On the other hand, an equilibrium is established for all gases on the surface of the bubble in the sense of equality of the partial pressures of each gas in solution and in the form of a free gas. And this means that the partial pressures of dissolved gases in water are equal to the corresponding values for saturation conditions, taking into account the partial pressures of gases in the free phase. Often, condition (1) is also written in a simplified form: ? Pi > pgz . ( 2 ) This approximation is acceptable for large depths when the Ratm is << pgz and the bubbles are not too small. For bubbles with a single-component composition, including for carbon dioxide bubbles, condition (1) reduces to the equality of the concentration of dissolved gas with the saturating concentration of this gas in water at a pressure of Ratm + pgz :
C = Snas(z) . ( 3 ) In turn, the Snac values are calculated using the Ostwald solubility coefficients ? for a given gas in water. The application of Henry's law, when the molar saturation concentration is calculated using Henry coefficients, is generally valid only for low pressures. However, at a water temperature of up to 30 °C and a pressure of up to 200 atm, the dependence of the molar saturating concentration of CO2 dissolved in water on pressure can still be considered linear [10]. Therefore, for the entire depth range of the Black Sea, the data in Table 1 can be used to estimate the Sna(z) by linear dependence. Accordingly, for a depth of 1000 m, we obtain a solubility of the order of 200 g /l. Thus, in its pure form, a limnological type disaster in volcanic lakes with carbon dioxide emissions cannot occur in the Black Sea. But something similar can happen in a small part of its water area in the event of a catastrophic eruption of underwater volcanoes, when bubbles of free gas reach the surface of the water before they completely dissolve. This does not require oversaturation of water with dissolved gases. Methane emissions at the bottom of the Black Sea are usually associated with mud volcanoes, a significant number of which are found near the coast of Crimea (Fig. 1) and on its shores in the Kerch region. In general, mud volcanism is characteristic of the Alpine-Himalayan geosynclinal belt. During the eruption of mud volcanoes, a significant amount of gases is emitted, among which methane and carbon dioxide usually predominate (Fig. 2). 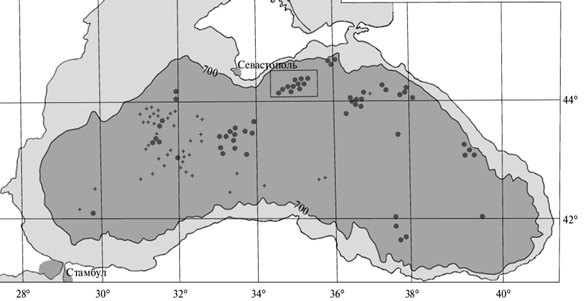 Fig. 1. Mud volcanoes at the bottom of the Black Sea [7]. The concentration of dissolved methane in the Black Sea is much less saturating. In deep waters, it is up to 0.02 mmol/l, which is an order of magnitude less than its concentration in Lake Nyos and three orders of magnitude less than in Lake Kivu (Table 2). However, due to the relatively low solubility of methane in water (it is approximately equal to the solubility of oxygen), methane bubbles are able to overcome significant the depth interval. And as additional components of the gas mixture, together with methane, carbon dioxide and hydrogen sulfide can thus enter the atmosphere. Although the release of methane itself poses a serious danger due to the formation of an explosive methane-air mixture. 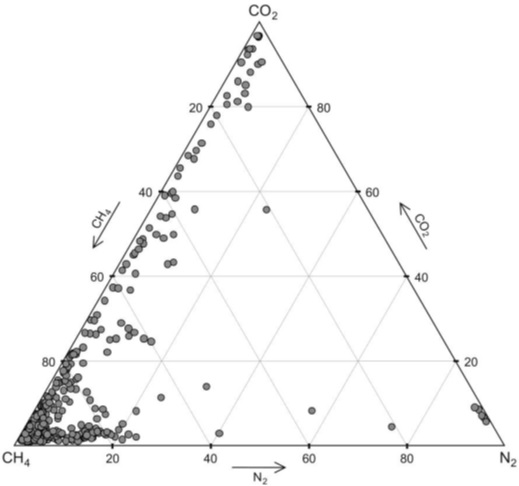 Fig. 2. Gas composition of mud volcano emissions [2]. Conclusion It should be noted that for mud volcanoes, as for ordinary volcanoes, the frequency of action is also characteristic. But it is more difficult to predict the next catastrophic release of gases in this case, since it is not preceded by a gradual increase in the concentration of dissolved gases in deep waters. It can only be noted that as the water temperature in the Black Sea increases due to climate change, methane gas hydrate reserves at the bottom of the sea will begin to decompose, which will also be accompanied by jet gas release, including through mud volcanoes. At the same time, methane can reach the surface from depths of no more than 900 m [1].
References
1. Artemov, Yu.G., Egorov, V.N., Gulin, S.B., & Polikarpov, G.G. (2013). New channels for jet methane discharge in the Sorokina depression in the deep-water part of the Black Sea. Marine Ecological Journal, 12(4), 27-36.
2. Bondarenko, D.D., Ershov, V.V. (2019). Gas geochemistry of mud volcanoes in connection with the forecast of oil and gas content of the earth's interior. Izvestia of Universities. North Caucasus region. Natural Sciences, 2, 41-46.
3. Degterev, A.Kh. (2017). The influence of hydrate formation on the manifestation of free gas releases of methane at the bottom of reservoirs. Geology and Geophysics, 58(9), 1388–1393.
4. Krasnova, E.D. (2021). Ecology of meromictic lakes in Russia. 1. Coastal marine reservoirs. Water resources, 48(3), 323-333.
5. Losyuk, G.N., Kokryatskaya, N.M., & Krasnova, E.D. (2021). Sulfide contamination of coastal lakes at different stages of isolation from the White Sea. Oceanology, 61(3), 401 – 412.
6. Reshetnyak, O.S., & Nikanorov, A.M. (2018). Hydrochemistry and protection of water resources. Rostov-on-Don: Publishing House of Southern Federal University.
7. Shnyukov, E.F. (2013). Mud volcanoes of the Black Sea as a search sign of methane gas hydrates. Lithology and minerals, 2, 114-121.
8. Shnyukov, E.F., Pasynkov, A.A., Lyubitsky, A.A. and others. (2010). Mud volcanoes on the Kerch section of the shelf and continental slope of the Black Sea. Geology and minerals of the World Ocean, 3, 28-36.
9. Barrenbold, F., Boehrer, B., Grilli, R., Mugisha, A. (2020). No increasing risk of limnic eruption at lake Kivu: Intercomparision stady reveals gas concentrations close to steady state. PLoS ONE, 8, 1-14.
10. Duan, Z., Sun, R. (2003). An improved model calculating CO2 solibility in pure water and aqueous NaCl solutions from 273 to 533 K and from 0 to 2000 bar. Chemical Geology, 193, 257-271.
11. Folch, A., Barcons, J., Kozono, T., & Costa, A. (2017). High-resolution modelling of atmospheric dispersion of dense gas using TWODEE-2.1: application to the 1986 Lake Nyos limnic eruption. Natural Hazards and Earth system Sciences, 17(6), 861-879.
12. Kling, G.W., Evans, W.C., & Tuttle, M.L. (1991). A comparative view of Lake Nyos and Monoun, Cameroon, West Africa. Internationale Vereinigang fur theoretische und angewandle Limnologie: Verhandlungen, 1102-1105.
13. Kozono, T., Kusakabe, M., Yoshida, Y. et al. (2016). Numerical assessment of the potential for future limnic eruptions at lakes Nyos and Monoun, Cameroon, based on regular monitoring data. Geological Society London Special Publication, 1-14.
14. Manirambona, E., Aaebisi, J.A., & Lucero-Prisno, III.E. (2021). Volcanic and limnic eruption: a potential threat to one health. PAMJ – One Health, 6(6), 1-5.
15. Zhang, Y. (1996). Dynamics of CO2 – driven lake eruptions. Letters to Nature, 379, 1-3.
Peer Review
Peer reviewers' evaluations remain confidential and are not disclosed to the public. Only external reviews, authorized for publication by the article's author(s), are made public. Typically, these final reviews are conducted after the manuscript's revision. Adhering to our double-blind review policy, the reviewer's identity is kept confidential.
The list of publisher reviewers can be found here.
The subject of the study, according to the author, is the assessment of the risk of degassing of the Black Sea such as the limnological disaster on Lake Nyoss using various calculation formulas. Research methodology based on the analysis of the article, it can be concluded that the methods of literary analysis, comparative characteristics of geographical objects and processes, the cartographic method, the method of diagramming are used. The relevance of the topic raised after two well-known disasters related to the release of dissolved carbon dioxide by volcanic lakes in Africa in the mid-1980s, the problem of the potential danger of meromictic reservoirs is very relevant. The research of the author of the article helps to understand the mechanism of the largest reservoir with a difficult vertical exchange - the Black Sea in case of changing weather and climatic conditions. The scientific novelty lies in the attempt of the author of the article, based on the research conducted, as the water temperature in the Black Sea increases due to climate change, methane gas hydrate reserves at the bottom of the sea will begin to decompose, which will also be accompanied by jet gas release, including through mud volcanoes, while methane can reach the surface from depths of no more than 900 M. Style, structure, content the style of presentation of the results is quite scientific. The article is provided with rich illustrative material reflecting the process of degasification of seawater and the release of gases from mud volcanoes, as well as the release of gas as a result of dinitrophication and rotting of organic residues in conditions of various depths of the Black Sea. The tables and graphs are illustrative, although, in our opinion, it would be necessary to outline the prospects for the use of methane gas hydrate, the volume of which is estimated at 25 trillion cubic meters, which suggests a high energy attractiveness of such a process. It was worth paying attention to exogenous factors affecting the degassing process, the use of calculation formulas depending on storage facilities, the degree of contact with the surrounding environment in the sea-atmosphere system, volumes and dynamics of temperature changes, thermal conductivity and heat capacity of the volda, and so on. The author considered the degassing of carbon dioxide, methane, but did not mention the problem of hydrogen sulfide degassing. Like other gases, hydrogen sulfide is an energy raw material, at the same time it can serve as a source of enormous environmental consequences, up to a catastrophe as a result of mixing of various layers of water in the Black Sea. The bibliography is very comprehensive for the formulation of the issue under consideration, but does not contain references to normative legal acts. The appeal to the opponents is presented in identifying the problem at the level of available information obtained by the author as a result of the analysis. Conclusions, the interest of the readership in the conclusions there are generalizations that allow us to apply the results obtained. The target group of information consumers is not specified in the article.
Link to this article
You can simply select and copy link from below text field.
|
|